1.
Basic Mechanics Kits- Description: These kits typically include components like gears, pulleys, levers, and inclined planes. They are used to demonstrate fundamental mechanical principles such as force, torque, motion, and energy transfer.
- Common Uses: Educational demonstrations, basic experiments in statics and dynamics.
- Tools and Components:
- Gears (spur, bevel, worm)
- Pulleys and belts
- Levers and fulcrums
- Inclined planes
- Springs and weights
- Ropes and strings
- Force gauges
- Screw sets and nuts
2.
Kinematics and Dynamics Kits- Description: Kits designed to study the motion of mechanisms, including linkages, cams, gears, and various types of motion converters (linear to rotational, etc.).
- Common Uses: Analysis of motion, design of mechanical linkages, study of velocity and acceleration in mechanical systems.
- Tools and Components:
- Linkages (four-bar, slider-crank)
- Cams and cam followers
- Gears and gear trains
- Shafts and axles
- Bearings
- Rotary encoders
- Stopwatches
- Protractors and rulers
3.
Thermodynamics and Heat Transfer Kits- Description: These kits may include components like heat exchangers, thermocouples, and various setups to study conduction, convection, and radiation. They are used to demonstrate and experiment with the principles of thermodynamics and heat transfer.
- Common Uses: Demonstrating laws of thermodynamics, studying energy conversion processes, heat transfer experiments.
- Tools and Components:
- Heat exchangers
- Thermocouples and thermometers
- Insulating materials
- Heating elements (electric heaters, Bunsen burners)
- Calorimeters
- Pressure gauges
- Fans and blowers (for convection experiments)
- Radiation plates and sensors
4.
Fluid Mechanics Kits- Description: Kits that include components like pumps, turbines, pipes, and flow meters. They are used to study fluid flow, pressure, and fluid-structure interactions.
- Common Uses: Demonstrating Bernoulli’s principle, studying laminar and turbulent flow, analyzing fluid systems.
- Tools and Components:
- Pumps (centrifugal, positive displacement)
- Flow meters (Venturi, orifice plate, rotameter)
- Piping and tubing
- Manometers and pressure gauges
- Valves (gate, ball, check)
- Turbines (small-scale)
- Nozzles and diffusers
- Fluid reservoirs
5.
Robotics Kits- Description: These kits typically include motors, sensors, controllers, and structural components to build and program robots. They may also come with software for simulation and control.
- Common Uses: Designing and programming robots, studying kinematics, automation, and control systems.
- Tools and Components:
- Servo motors and DC motors
- Microcontrollers (Arduino, Raspberry Pi)
- Sensors (ultrasonic, infrared, light)
- Wheels and tracks
- Structural components (beams, plates, fasteners)
- Breadboards and jumper wires
- Battery packs and power supplies
- Programming cables
6.
Control Systems Kits- Description: Kits that provide components such as servos, controllers, sensors, and feedback devices to study control theory and implement control systems.
- Common Uses: PID controller experiments, stability analysis, automation tasks.
- Tools and Components:
- PID controllers
- Servos and actuators
- Sensors (temperature, pressure, position)
- Control loops (analog and digital)
- Microcontrollers or PLCs
- Oscilloscopes
- Signal generators
- Relay modules and switches
7.
Vibration and Dynamics Kits- Description: Kits that allow the study of vibrational characteristics of mechanical systems. They typically include components for generating and measuring vibrations, such as accelerometers, mass-spring systems, and damping materials.
- Common Uses: Studying natural frequencies, damping, and resonance in mechanical structures.
- Tools and Components:
- Accelerometers
- Vibration motors
- Springs and masses
- Damping materials (rubber pads, foam)
- Frequency counters
- Shakers (vibration generators)
- Signal analyzers
- Tunable mass dampers
8.
Materials Science Kits- Description: These kits may include samples of different materials, testing equipment (like hardness testers), and tools for analyzing material properties such as tensile strength, elasticity, and fracture toughness.
- Common Uses: Material testing, failure analysis, studying the properties of metals, polymers, and composites.
- Tools and Components:
- Universal Testing Machine (UTM) components
- Hardness testers (Rockwell, Brinell)
- Sample materials (metals, polymers, composites)
- Microscopes (optical)
- Strain gauges
- Impact testers
- Metallurgical mounts
- Fracture analysis tools
9.
Machine Design Kits- Description: Kits that include components like gears, shafts, bearings, and fasteners to design and test mechanical assemblies and systems. They may also include CAD files for 3D modeling.
- Common Uses: Prototyping mechanical systems, studying load distribution, stress analysis, and design optimization.
- Tools and Components:
- Gears (spur, helical, worm)
- Shafts and keys
- Bearings (ball, roller)
- Fasteners (bolts, nuts, washers)
- Sprockets and chains
- Couplings (rigid, flexible)
- Belts and pulleys
- Tolerance gauges and calipers
10.
Energy Conversion Kits- Description: Kits focused on the principles of energy conversion, including components like solar panels, wind turbines, and small-scale engines. They are used to study and experiment with converting energy from one form to another.
- Common Uses: Experiments in renewable energy, studying thermodynamic cycles, efficiency analysis.
- Tools and Components:
- Small solar panels
- Wind turbine models
- Electric generators and motors
- Thermoelectric generators
- Batteries and energy storage devices
- Inverters and converters
- Energy meters and sensors
- Thermal engines (Stirling, steam)
11.
CNC and Machining Kits- Description: Small-scale CNC kits that include a computer-controlled milling machine or lathe, along with the necessary software and tooling. These kits are used to introduce students and hobbyists to CNC machining.
- Common Uses: Prototyping, precision machining, learning CNC programming.
- Tools and Components:
- CNC milling machine or lathe
- Cutting tools (end mills, drill bits)
- Tool holders and collets
- Workholding devices (clamps, vises)
- Coolant system (basic)
- CAD/CAM software
- G-code generator
- Safety glasses and gloves
12.
Additive Manufacturing (3D Printing) Kits- Description: Kits that include a 3D printer and materials like PLA, ABS, or resin. These kits are used for prototyping and studying the principles of additive manufacturing.
- Common Uses: Rapid prototyping, custom part manufacturing, studying additive manufacturing processes.
- Tools and Components:
- 3D printer (FDM or resin)
- Filament or resin material
- Build plate and adhesives
- Nozzles and extruders
- CAD software
- Calibration tools (bed levelers, gauges)
- Scrapers and cutters (for part removal)
- UV light (for resin curing)
13.
Hydraulics and Pneumatics Kits- Description: These kits include pumps, cylinders, valves, and actuators to demonstrate hydraulic and pneumatic systems. They are used to study the principles of fluid power and control.
- Common Uses: Building and controlling fluid power systems, studying hydraulic and pneumatic actuators.
- Tools and Components:
- Hydraulic pumps and cylinders
- Pneumatic actuators and valves
- Hoses and fittings
- Pressure gauges
- Flow control valves
- Reservoirs
- Compressors
- Control panels (manual or automated)
14.
Wind and Solar Energy Kits- Description: Kits designed to explore renewable energy technologies, often including small-scale solar panels, wind turbines, and associated measurement tools.
- Common Uses: Experiments in renewable energy generation, studying energy efficiency, and sustainability.
- Tools and Components:
- Small wind turbine blades and generator
- Solar panels
- Inverters and charge controllers
- Battery packs
- Energy meters
- Load resistors (for testing)
- Mounting hardware
- Simulation software (optional)
15.
Automotive Engineering Kits- Description: Kits that simulate automotive systems, including components like small engines, transmissions, braking systems, and suspension models.
- Common Uses: Studying vehicle dynamics, engine performance, and automotive systems design.
- Tools and Components:
- Small-scale engines (gasoline, electric)
- Transmission models
- Suspension systems (springs, dampers)
- Braking systems (disk, drum)
- Steering mechanisms Models
- Differential models
- Diagnostic tools (multi-meters, OBD scanners)
- Fuel and exhaust systems (carburetors, mufflers)
These kits are valuable resources for hands-on learning, experimentation, and the application of mechanical engineering principles in real-world scenarios.
there is huge gap between these two and thats actually leads to unemployment in engineering fields although there are lots of scope to work as an engineer but still lots of vacancy is not getting fulfilled and people get unemployed even though they got degree.
Academic Engineering and Industrial Engineering represent two distinct spheres within the broader field of engineering, each with its own focus, goals, and approaches. Here's a breakdown of the key differences between the two:
1.
Purpose and FocusAcademic Engineering:
- Purpose: Focuses on the theoretical, scientific, and educational aspects of engineering. It aims to expand knowledge through research, teaching, and the development of new theories and methodologies.
- Focus: In-depth exploration of fundamental principles, mathematical modeling, simulations, and theoretical analysis. Academic engineers often work on advancing the frontiers of knowledge in their field.
Industrial Engineering:
- Purpose: Concerned with applying engineering principles to optimize processes, systems, and organizations within industries. The goal is to improve efficiency, productivity, and quality in real-world applications.
- Focus: Practical implementation of engineering knowledge in manufacturing, logistics, supply chain management, and operations. Industrial engineers work to solve practical problems and enhance industrial processes.
2.
Research and DevelopmentAcademic Engineering:
- Research: Typically involves basic or fundamental research aimed at discovering new knowledge without immediate commercial application. Academic research often leads to publications in scientific journals and conferences.
- Development: May involve the development of new theories, algorithms, or techniques that contribute to the academic body of knowledge. Development is usually more conceptual and less concerned with immediate industrial application.
Industrial Engineering:
- Research: Focuses on applied research that directly addresses industry-specific challenges. Research is often driven by the need to solve specific problems or improve existing processes within a company or sector.
- Development: Involves the design, implementation, and optimization of processes, systems, or products. Development is closely tied to practical outcomes and often leads to new products, improved systems, or increased efficiency.
3.
Application of KnowledgeAcademic Engineering:
- Application: Knowledge is applied primarily in an educational setting (teaching) or within research labs. The results may influence industrial practices but are often several steps removed from direct application.
- Outcome: Contributes to the theoretical foundation and future technologies. The impact on industry is typically long-term, as academic findings may take years to be applied.
Industrial Engineering:
- Application: Directly applies engineering principles to solve problems within industry. Industrial engineers work on projects that have immediate or short-term impacts on the company’s operations.
- Outcome: Results in tangible improvements in processes, cost savings, enhanced productivity, and quality within a relatively short timeframe.
so these how things get not resolved by engineering passed out student to get job on desired role in industry but by following steps we might resolve this issues.
Bridging the gap between academic engineering and industrial engineering can enhance innovation, improve practical outcomes, and ensure that theoretical advances translate effectively into real-world applications. Here are some strategies to help fulfill this gap:
1.
Collaborative Research Initiatives- Industry-Academia Partnerships: Encourage collaborations between universities and industry to work on joint research projects. Companies can provide practical problems, while academic researchers can offer theoretical insights and advanced methodologies.
- Sponsored Research: Industries can sponsor research at universities focused on specific challenges they face. This ensures that academic research is aligned with industrial needs.
2.
Internships and Industry Placements- Academic Internships: Encourage students and faculty to engage in internships or sabbaticals in industrial settings. This exposes academics to real-world challenges and gives them practical insights that can inform their research.
- Industry Fellowships: Create fellowships where industry professionals can spend time in academic institutions, sharing their practical experience and learning about the latest research developments.
3.
Curriculum Integration- Problem-Based Learning: Integrate real-world industrial problems into the curriculum. Students can work on these as part of their coursework, bridging the gap between theoretical learning and practical application.
- Guest Lectures and Workshops: Invite industry professionals to give guest lectures or conduct workshops, bringing practical insights into the academic setting.
4.
Applied Research and Development Centers- University Research Centers: Establish research centers within universities that focus specifically on applied research. These centers can serve as hubs for industry-academic collaboration, focusing on solving practical engineering problems.
- Technology Transfer Offices: Universities can set up offices dedicated to technology transfer, helping to commercialize academic research and bring it to the market.
5.
Dual Career Paths- Industry-Academic Roles: Encourage professionals to pursue dual careers in both academia and industry. For example, an academic might spend part of their time conducting research at a university and part of their time consulting for industry.
- Adjunct Professorships: Industry professionals can be appointed as adjunct professors, allowing them to contribute to academic teaching and research while remaining active in the industry.
6.
Collaborative Funding Opportunities- Joint Funding Programs: Governments and funding bodies can create programs that specifically support projects involving both academic institutions and industrial partners.
- Innovation Grants: Provide grants for collaborative projects that require academic research to be applied in an industrial context. This encourages both parties to work together toward a common goal.
7.
Knowledge Exchange Platforms- Conferences and Workshops: Organize events that bring together academics and industry professionals to share knowledge, discuss challenges, and explore collaborative opportunities.
- Online Platforms: Create online forums, databases, and platforms where industry and academia can share research findings, case studies, and best practices.
8.
Curriculum Development and Continuous Education- Industry-Driven Curriculum: Involve industry representatives in curriculum development to ensure that academic programs are aligned with current industry needs and trends.
- Continuous Education Programs: Offer continuing education and professional development programs that allow industry professionals to stay updated with the latest academic research and engineering advancements.
9.
Feedback Loops- Post-Implementation Feedback: After implementing academic research in an industrial setting, gather feedback on its effectiveness. This feedback can inform future research and help academics understand the practical challenges of implementation.
- Industry Advisory Boards: Establish industry advisory boards for academic departments. These boards can provide insights into current industry needs and guide academic research priorities.
10.
Innovation Ecosystems- Incubators and Accelerators: Universities can establish incubators and accelerators that support the commercialization of academic research. These can provide resources, mentorship, and connections to industry.
- Spin-Off Companies: Encourage the creation of spin-off companies from academic research, which can directly apply innovative ideas in a commercial context.
11.
Case Studies and Applied Research Publications- Document and Share Successes: Publish case studies and applied research papers that detail successful industry-academic collaborations. Sharing these success stories can inspire more partnerships.
- Applied Engineering Journals: Promote the creation or use of academic journals that focus specifically on applied engineering, where research findings are directly related to industrial applications.
By implementing these strategies, the gap between academic and industrial engineering can be significantly reduced, leading to more effective innovation and practical outcomes that benefit both academia and industry.
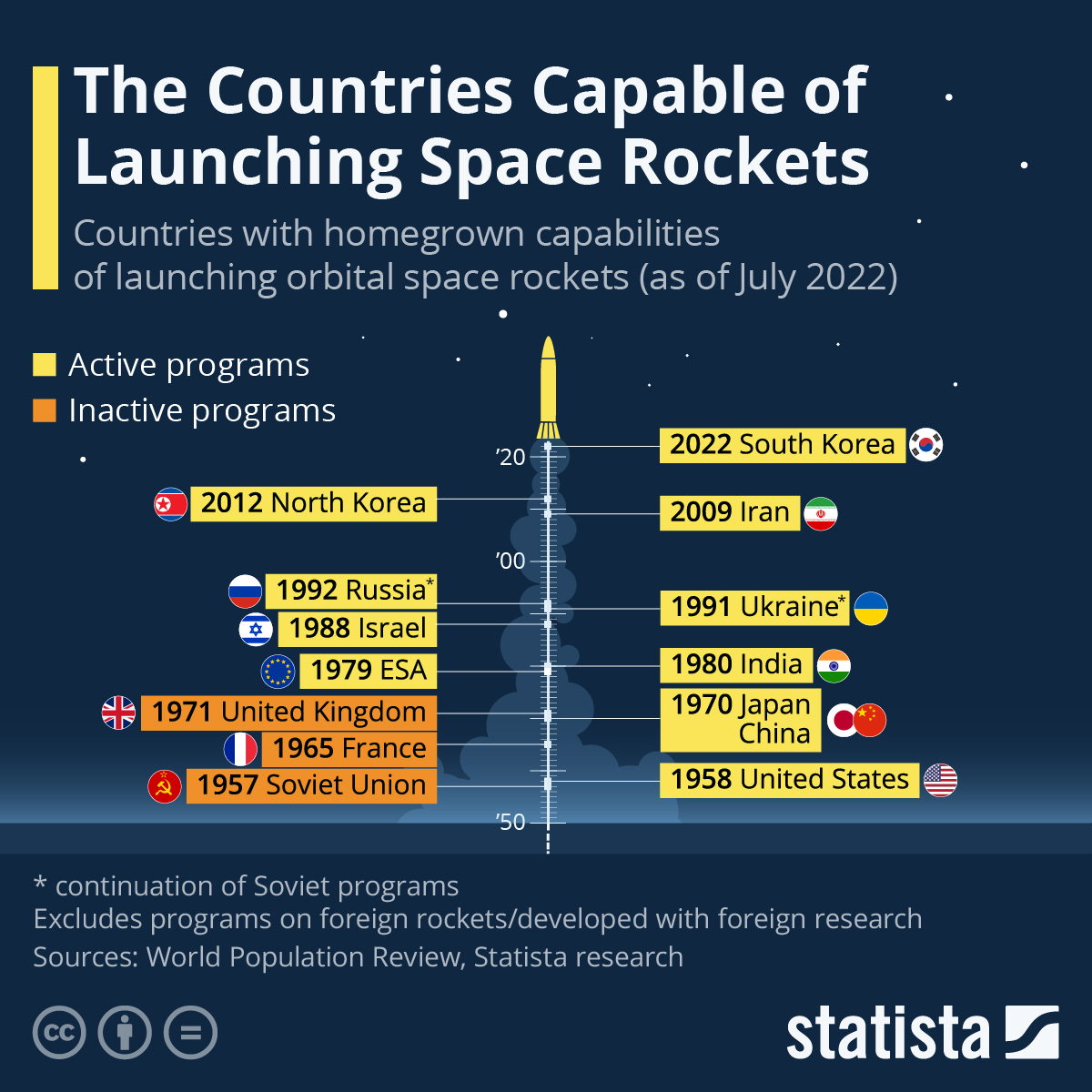
Several countries have developed their own rockets capable of launching payloads into space. These countries have invested in space programs that include the design, testing, and deployment of orbital rockets. Here is a list of countries that have successfully built and launched such rockets:
1.
United States- Notable Rockets: Saturn V, Space Shuttle, Falcon 9, Delta IV, Atlas V, SLS (Space Launch System)
- Space Agency: NASA (National Aeronautics and Space Administration)
- Private Companies: SpaceX, Blue Origin, United Launch Alliance (ULA)
2.
Russia (formerly the Soviet Union)- Notable Rockets: R-7 (Soyuz), Proton, Zenit, Angara
- Space Agency: Roscosmos (Russian Federal Space Agency)
3.
China- Notable Rockets: Long March series (Chang Zheng), including Long March 3B, Long March 5
- Space Agency: CNSA (China National Space Administration)
4.
France (and the European Union through ESA)- Notable Rockets: Ariane series (Ariane 5, Ariane 6)
- Space Agency: CNES (National Centre for Space Studies) and ESA (European Space Agency)
5.
Japan- Notable Rockets: H-IIA, H-IIB, Epsilon
- Space Agency: JAXA (Japan Aerospace Exploration Agency)
6.
India- Notable Rockets: PSLV (Polar Satellite Launch Vehicle), GSLV (Geosynchronous Satellite Launch Vehicle)
- Space Agency: ISRO (Indian Space Research Organisation)
7.
Israel- Notable Rockets: Shavit
- Space Agency: ISA (Israel Space Agency)
8.
Iran- Notable Rockets: Safir, Simorgh
- Space Agency: ISA (Iranian Space Agency)
9.
North Korea- Notable Rockets: Unha series (derived from Taepodong-2)
- Space Agency: NADA (National Aerospace Development Administration)
10.
South Korea- Notable Rockets: Naro-1, KSLV-II (Nuri)
- Space Agency: KARI (Korea Aerospace Research Institute)
11.
United Kingdom- Notable Rockets: Black Arrow (historical; UK no longer has an active national rocket program but is involved in international collaborations)
- Space Agency: UKSA (UK Space Agency)
12.
Brazil- Notable Rockets: VLS-1 (program was developed but not fully successful; Brazil is still active in space technology development)
- Space Agency: AEB (Brazilian Space Agency)
13.
Ukraine- Notable Rockets: Zenit, Tsyklon (inherited from Soviet-era technologies, Ukraine continues to develop space technologies)
- Space Agency: SSAU (State Space Agency of Ukraine)
Emerging Space Nations
In addition to the countries listed above, several other nations are actively developing or have developed rocket technology with varying degrees of success, including:
- Turkey: Developing the Gokturk series of rockets.
- United Arab Emirates: Partnering with other nations, with ambitions for indigenous rocket development.
- Australia: Developing the capability for launching small satellites.
Conclusion
These countries have made significant investments in their space programs, developing the necessary infrastructure, technology, and expertise to build and launch rockets capable of reaching space. The list is evolving as more nations seek to develop or expand their space capabilities, reflecting the growing global interest in space exploration and satellite deployment.